Tornado formation
In our NSF funded research, we use
3D vorticity budgets as well as Truesdell's vorticity formula along air-parcel trajectories
and apply it to more or less idealized numerical simulations.
This way the different sources of rotation can be isolated with way. Some
highlights of our research are the recognition that the
mechanism by which the initial near-surface rotation is established is different from
the mechanism that maintains tornado.
Initial seed rotation
Initially, it is air that has traveled through the forward flank region of the storm that
contributes to rotation at the surface. This air
has been cooled and thus descended to the ground, whereby it has acquired horizontal spin
at the periphery of the downdraft. This horizontal spin is tipped upward while the air
is descending, a mechanism first identified by R. Davies-Jones and H. Brooks in the 1990s.
This mechanism is described in detail in Dahl et al. (2014), and is shown in Fig. 1.
Fig. 1: The baroclinically produced vorticity vector (blue arrows) is tipping upward while
the air is descending. The abscissa represents the horizontal distance in meters, and the ordinate
represents height in meters.
Vortex formation
In a recent study
we show that there is a transition from this downdraft mechanism toward
what we called the "in-and-up" mechanism, by which horizontal near-surface vorticity is tilted
into the vertical abruptly at the base of the tornado. A conceptual model of this transition
mechanism is shown in Fig. 2.
Fig. 2: Schematic of the transition from the downdraft mechanism to the "in-and-up"
mechanism. The blue arrows represent the vorticity vector, and the
red regions represent the vortex patch. From Fischer and Dahl (2022).
The main vorticity source at this stage is likely related to surface drag, although
it seems that any vorticity can be utilized (baroclinically produced vorticity, and
even ambient vorticity!). Ongoing research is focused on identifying e.g., the role of
ambient vorticity in maintaining the mature tornado.
Vortex intensification during tornadogenesis
While is is well-known that some "seed" rotation at the ground needs to be amplified via
horizontal convergence in order to make a tornado, it seems that a lot more is going on.
In particular, the seed "rotation" often is manifest as an elongated "streamer" of vertical vorticity
that first needs to become axisymmetrized (i.e., a vortex needs to form out of the
region of vertical vorticity; this is not dissimilar from the rolling-up of a vorticity
band due to shear instability - see here for more information). Figure 3 shows the symmetrization process.
Fig. 3: Organization of an elongated vortex patch during the tornadogenesis
process. The left column shows the vertical-vorticity field, and the
right column shows the kinematical vorticity number. From Dahl (2020).
Once the vortex forms, the central pressure falls, and the transition to the in-and-up mechanism
occurs. It seems that rapid amplification may occur via a feedback between the central pressure drop,
upward tilting of horizontal vortex lines, vertical stretching, etc. We are currently analyzing this
mechanism using a global instability analysis in collaboration with
the FLOW Group led by Prof.
Oberleithner at the Technical University of Berlin.
Synoptic- and mesoscale dynamics
In a previous research project, we used Q-vector diagnostics to analyze the development
of prefrontal convergence lines over western Europe. These lines occur several 100 miles ahead of the
cold front and frequently promote thunderstorm development. We found that this development
is tied to the elevated mixed layer (EML), a plume of hot and unstable air originating from the
elevated terrain of the Iberian peninsula and northern Africa. A sketch of the development
of the line (depicted as dashed line) relative to the EML (shaded blush region) is shown in Fig. 4.
Fig. 4: Schematic of how the elevated mixed layer (blush shading) is integrated into the
warm sector, leading to frontogenesis and rising motion ahead of the cold front.
From Dahl and Fischer (2016).
For more information about our research, feel free to browse our publications
below (most papers are freely accessible).
List of peer-reviewed journal articles
(Group members are listed in boldface)
[21] Dahl, J. M. L., 2024: The develpment of simulated dust-devil-like vortices. J. Atmos. Sci., 81, 1883-1899.
Link
[20] Fischer, J., J. M. L. Dahl, B. E. Coffer, J. Lesak Houser, P. M. Markowski, M. D. Parker, C. C. Weiss, and A. Schueth, 2024: Supercell tornadogenesis: Recent progress in our state of
understanding. Bull. Amer. Meteor. Soc., 105,E1084-E1097. Link
[19] Dahl, J. M. L. and J. Fischer, 2023: On the origins of vorticity in a simulated tornado-like vortex.
J. Atmos. Sci., 80, 1361-1380. Link
[18] Fischer, J. and J. M. L. Dahl, 2023:
Supercell-external storms and boundaries acting as catalysts for tornadogenesis.
Mon. Wea. Rev., 151, 23-38. Link
[17] Fischer, J. and J. M. L. Dahl, 2022:
Transition of near-ground vorticity dynamics during tornadogenesis.
J. Atmos. Sci., 79, 467-483. Link
[16] Dahl, J. M. L., 2021:
Centrifugal waves in tornado-like vortices: Kelvin's solutions and their
applications to multiple-vortex development and vortex breakdown.
Mon. Wea. Rev., 149, 3173-3216. Link
[15] Schueth, A., C. Weiss, and J. M. L. Dahl, 2021:
Comparing observations and simulations of the streamwise vorticity
current and the forward flank convergence boundary in a supercell storm. Mon. Wea. Rev., 149, 1651-1671.
Link
[14] Fischer, J. and J. M. L. Dahl, 2020:
The relative importance of updraft and cold pool characteristics on supercell tornadogenesis in highly idealized simulations. J. Atmos. Sci., 77, 4089-4107. Link
[13] Boyer, C. H. and J. M. L. Dahl, 2020:
The mechanisms responsible for large near-surface vertical vorticity within simulated
supercells and quasi-linear storms.
Mon. Wea. Rev., 148, 4281-4297. Link"
[12] Dahl, J. M. L., 2020:
Near-surface vortex formation in supercells from the perspective of vortex patch dynamics.
Mon. Wea. Rev., 148, 3533–3547. Link
[11] Vande Guchte, A. and J. M. L. Dahl, 2018:
Sensitivities of parcel trajectories beneath the lowest scalar model level of a Lorenz vertical grid.
Mon. Wea. Rev., 146, 1427-1435. Link
[10] Coffer, B. E., M. D. Parker, J. M. L. Dahl, L. J. Wicker, and A. J. Clark, 2017:
Volatility of tornadogenesis: An ensemble of simulated nontornadic and tornadic supercells in VORTEX2 environments.
Mon. Wea. Rev., 145, 4605-4625. Link
[9] Dahl, J. M. L., 2017:
Tilting of horizontal shear vorticity and the development of updraft rotation in supercell thunderstorms.
J. Atmos. Sci., 74, 2997-3020. Link
[8] Dahl, J. M. L. and J. Fischer, 2016:
The origin of western European warm-season prefrontal convergence lines.
Wea. Forecasting, 31, 1417-1431. Link
[7] Dahl, J. M. L., 2015:
Near-ground rotation in simulated supercells: On the robustness of the baroclinic mechanism.
Mon. Wea. Rev., 143, 4929-4942. Link
[6] Parker, M. D. and J. M. L. Dahl, 2015: Production of near-surface
vertical vorticity by downdrafts. Mon. Wea. Rev., 143, 2795-2816. Link
[5] Dahl, J. M. L., P. M. Markowski, 2014:
Comment on "Eliminating the major tornado threat in Tornado Alley."
Int. J. Mod. Phys. B, 28, 1475004. Link
[4] Dahl, J. M. L., M. D. Parker, and L. J. Wicker, 2014:
Imported and storm-generated near-ground vertical
vorticity in a simulated supercell.
J. Atmos. Sci., 71, 3027-3051. Link
[3] Dahl, J. M. L., M. D. Parker, and L. J. Wicker, 2012:
Uncertainties in trajectory calculations within
near-surface mesoscyclones of simulated supercells.
Mon. Wea. Rev., 140, 2959-2966. Link
[2] Dahl, J. M. L., H. Hoeller, and U. Schumann, 2011:
Modeling the flash rate of thunderstorms. Part II: Implementation.
Mon. Wea. Rev., 139, 3112-3124. Link
[1] Dahl, J. M. L., H. Hoeller, and U. Schumann, 2011:
Modeling the flash rate of thunderstorms.
Part I: Framework. Mon. Wea. Rev., 139, 3093-3111. Link
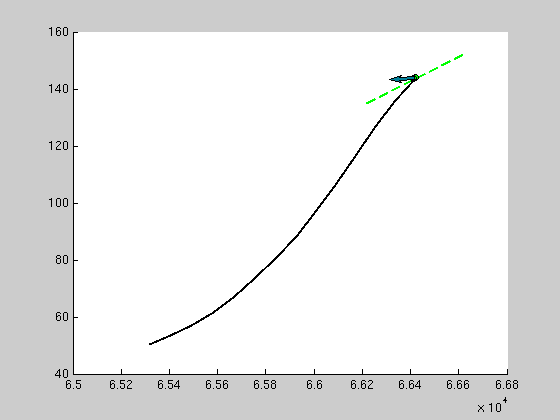
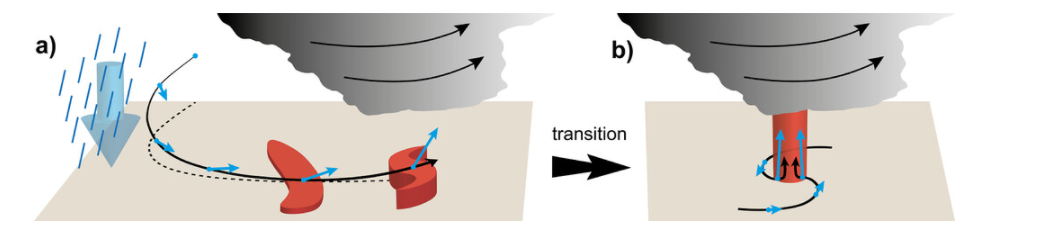
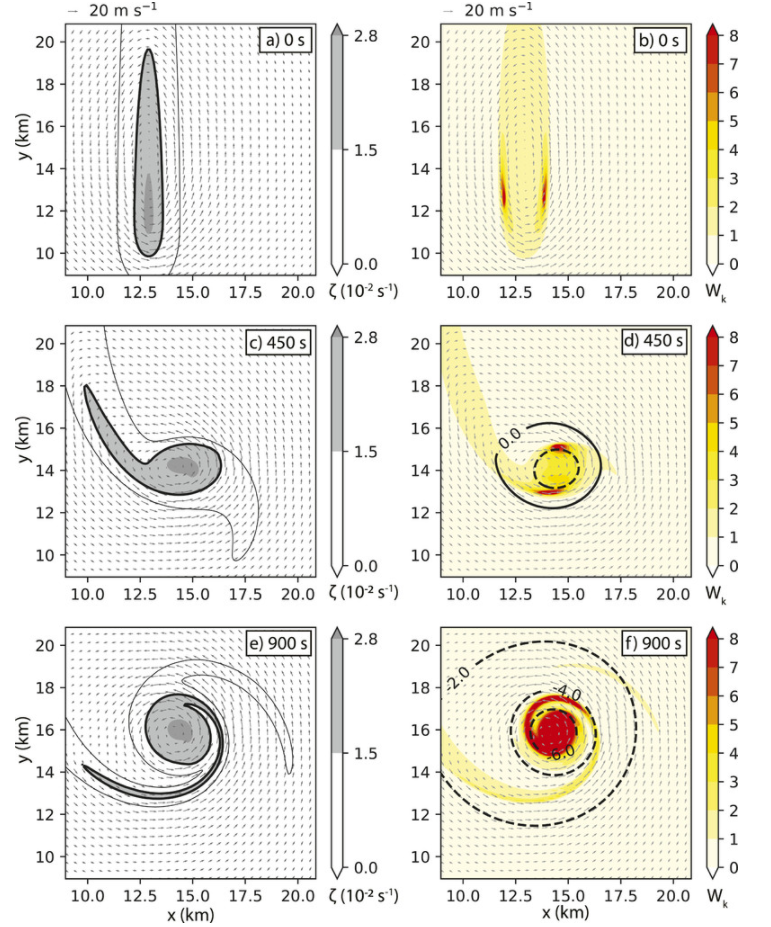
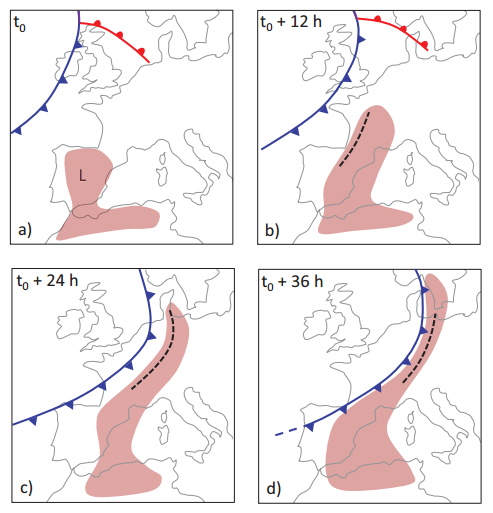
[20] Fischer, J., J. M. L. Dahl, B. E. Coffer, J. Lesak Houser, P. M. Markowski, M. D. Parker, C. C. Weiss, and A. Schueth, 2024: Supercell tornadogenesis: Recent progress in our state of understanding. Bull. Amer. Meteor. Soc., 105,E1084-E1097. Link
[19] Dahl, J. M. L. and J. Fischer, 2023: On the origins of vorticity in a simulated tornado-like vortex. J. Atmos. Sci., 80, 1361-1380. Link
[18] Fischer, J. and J. M. L. Dahl, 2023: Supercell-external storms and boundaries acting as catalysts for tornadogenesis. Mon. Wea. Rev., 151, 23-38. Link
[17] Fischer, J. and J. M. L. Dahl, 2022: Transition of near-ground vorticity dynamics during tornadogenesis. J. Atmos. Sci., 79, 467-483. Link
[16] Dahl, J. M. L., 2021: Centrifugal waves in tornado-like vortices: Kelvin's solutions and their applications to multiple-vortex development and vortex breakdown. Mon. Wea. Rev., 149, 3173-3216. Link
[15] Schueth, A., C. Weiss, and J. M. L. Dahl, 2021: Comparing observations and simulations of the streamwise vorticity current and the forward flank convergence boundary in a supercell storm. Mon. Wea. Rev., 149, 1651-1671. Link
[14] Fischer, J. and J. M. L. Dahl, 2020: The relative importance of updraft and cold pool characteristics on supercell tornadogenesis in highly idealized simulations. J. Atmos. Sci., 77, 4089-4107. Link
[13] Boyer, C. H. and J. M. L. Dahl, 2020: The mechanisms responsible for large near-surface vertical vorticity within simulated supercells and quasi-linear storms. Mon. Wea. Rev., 148, 4281-4297. Link"
[12] Dahl, J. M. L., 2020: Near-surface vortex formation in supercells from the perspective of vortex patch dynamics. Mon. Wea. Rev., 148, 3533–3547. Link
[11] Vande Guchte, A. and J. M. L. Dahl, 2018: Sensitivities of parcel trajectories beneath the lowest scalar model level of a Lorenz vertical grid. Mon. Wea. Rev., 146, 1427-1435. Link
[10] Coffer, B. E., M. D. Parker, J. M. L. Dahl, L. J. Wicker, and A. J. Clark, 2017: Volatility of tornadogenesis: An ensemble of simulated nontornadic and tornadic supercells in VORTEX2 environments. Mon. Wea. Rev., 145, 4605-4625. Link
[9] Dahl, J. M. L., 2017: Tilting of horizontal shear vorticity and the development of updraft rotation in supercell thunderstorms. J. Atmos. Sci., 74, 2997-3020. Link
[8] Dahl, J. M. L. and J. Fischer, 2016: The origin of western European warm-season prefrontal convergence lines. Wea. Forecasting, 31, 1417-1431. Link
[7] Dahl, J. M. L., 2015: Near-ground rotation in simulated supercells: On the robustness of the baroclinic mechanism. Mon. Wea. Rev., 143, 4929-4942. Link
[6] Parker, M. D. and J. M. L. Dahl, 2015: Production of near-surface vertical vorticity by downdrafts. Mon. Wea. Rev., 143, 2795-2816. Link
[5] Dahl, J. M. L., P. M. Markowski, 2014: Comment on "Eliminating the major tornado threat in Tornado Alley." Int. J. Mod. Phys. B, 28, 1475004. Link
[4] Dahl, J. M. L., M. D. Parker, and L. J. Wicker, 2014: Imported and storm-generated near-ground vertical vorticity in a simulated supercell. J. Atmos. Sci., 71, 3027-3051. Link
[3] Dahl, J. M. L., M. D. Parker, and L. J. Wicker, 2012: Uncertainties in trajectory calculations within near-surface mesoscyclones of simulated supercells. Mon. Wea. Rev., 140, 2959-2966. Link
[2] Dahl, J. M. L., H. Hoeller, and U. Schumann, 2011: Modeling the flash rate of thunderstorms. Part II: Implementation. Mon. Wea. Rev., 139, 3112-3124. Link
[1] Dahl, J. M. L., H. Hoeller, and U. Schumann, 2011: Modeling the flash rate of thunderstorms. Part I: Framework. Mon. Wea. Rev., 139, 3093-3111. Link